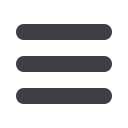
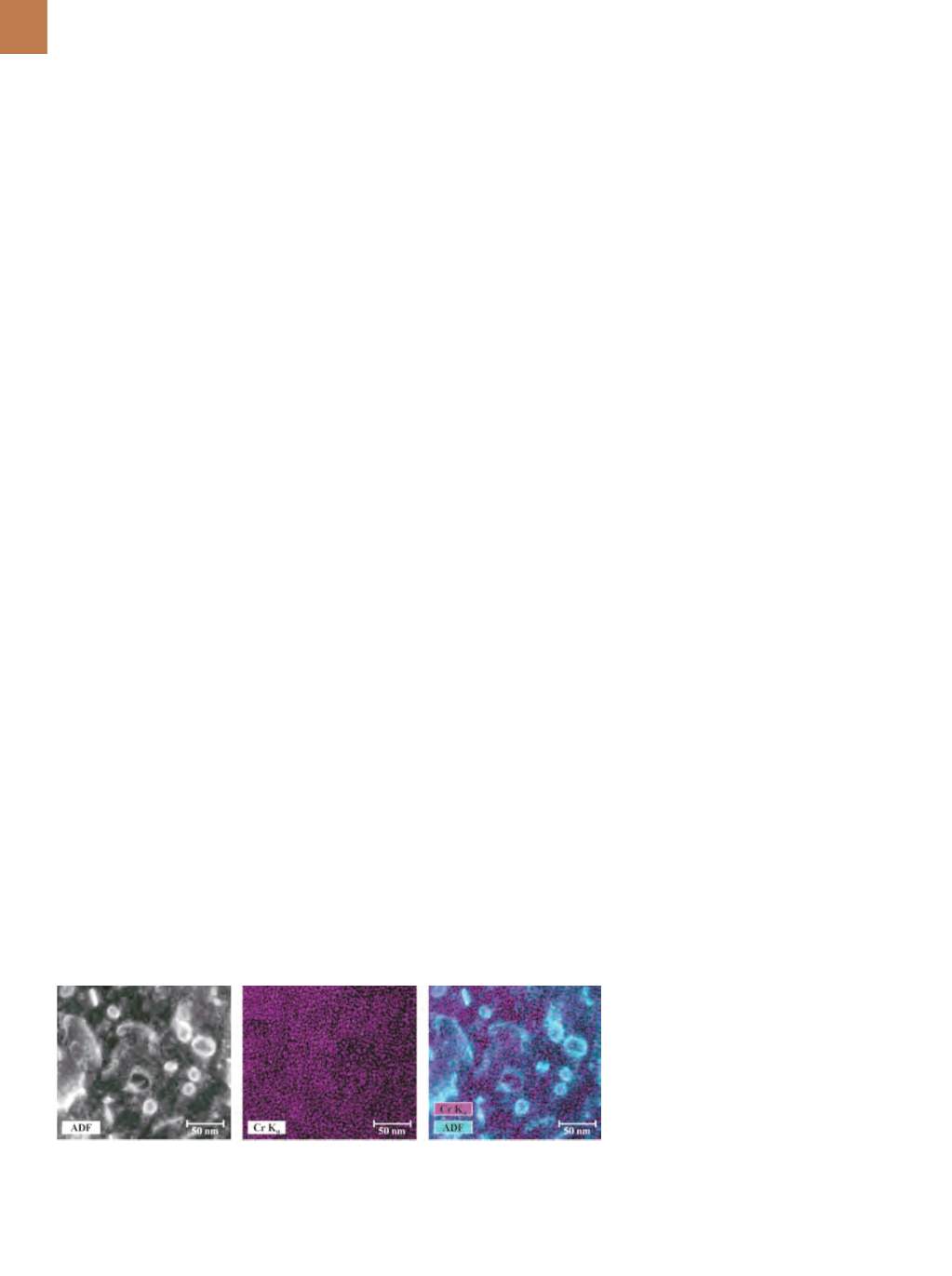
A D V A N C E D M A T E R I A L S & P R O C E S S E S | N O V E M B E R / D E C E M B E R 2 0 1 6
2 0
models used in the scattering analy-
sis. Artifacts of nuclear transmutation
resulting from neutron absorption are
observed in the generated time-of-flight
spectrum as manifested by peak ratios
that are inconsistent with expected
natural isotopic abundances of constit-
uent elements. In addition, peaks cor-
responding to transmutation products,
such as V and Mn, are also identified.
Due to the semi-coherency of
α
ʹ
particles in an
α
-ferrite matrix, conven-
tional diffraction contrast-based TEM
techniques are not useful for studying
the Cr-rich precipitates in this system,
necessitating the use of chemically
sensitive electron microscopy tech-
niques
[9]
. As such, energy dispersive
x-ray spectroscopy (EDS) was coupled
with STEM to acquire spectral images of
the precipitate microstructure. STEM/
EDS data collection was performed on
the FEI Talos F200X S/TEM located in
the LAMDA facility. Due to the high effi-
ciency of the FEI Talos system, care must
be taken when performing data analysis
as the energetic emissions of radioactive
decay from irradiated samples can be
easily detected along with the charac-
teristic x-rays, potentially muddling the
observed x-ray spectrum; this effect is
significantly reduced with FIB prepared
specimens over more traditional 3 mm
disc specimens. The x-ray maps were
collected concurrently with annular
dark field (ADF) images on the [111] zone
axis, allowing for simultaneous imaging
of dislocation loops
[10]
and collection
of EDS spectra in these materials. Fig-
ure 5 demonstrates that
α
ʹ precipitates
appear to nucleate homogenously in the
bulk material, with no bias for disloca-
tion loop or other defect sites.
THE FUTURE OF NUCLEAR
MATERIALS RESEARCH
Modern nuclear materials char-
acterization capabilities allow for an
in-depth, multifaceted investigation
of nanoscale precipitation events in
alloys, as demonstrated for
α
ʹ phase
precipitation in neutron-irradiated
Fe-Cr-Al alloys for accident-toler-
ant nuclear fuels applications. The
resulting detailed analysis of the
mechanisms and dependencies of pre-
cipitation in this system has informed
design decisions within the Fe-Cr-Al
alloy development program of the
DOE’s Advanced Fuels Campaign.
Generation II Fe-Cr-Al engineering
alloys with down-selected composi-
tions based on this work are currently
undergoing irradiation in HFIR to
study the effect of minor alloying ele-
ment additions on alloy radiation tol-
erance with the goal of recommending
a nuclear-grade Fe-Cr-Al LWR cladding
material in the near future.
Fe-Cr-Al alloys are just one of
many material systems currently being
developed for nuclear applications. For
example, SiC is being extensively inves-
tigated both as an alternative LWR clad-
ding material and for advanced TRISO
particle fuel for gas-cooled reactor tech-
nologies. Late-blooming phases (LBP)
that may embrittle reactor pressure
vessel (RPV) materials are also under
investigation in order to extend com-
mercial LWR operating licenses from
60 to 80 years. Additionally, improved
radiation tolerance of oxide-dispersion
strengthened (ODS) and nanostruc-
tured materials is being explored for
other in-core components.
Advancing nuclear materials tech-
nology and developing robust radi-
ation-tolerant alloys, ceramics, and
nuclear fuels is critical for both main-
taining and improving the existing com-
mercial LWR technologies in addition to
enabling the construction of next-gen-
eration reactor designs with even more
demanding environments for materials
performance. The modern characteri-
zation capabilities discussed here have
greatly facilitated high-quality charac-
terization research on nanoscale radia-
tion effects in materials. The continued
adoption of advanced characterization
techniques and equipment in addition
to ample access to this equipment by
nuclear materials researchers is antici-
pated to play a central role in enabling
informed materials development for
nuclear applications.
ACKNOWLEDGMENTS
Primary research funding was
sponsored by DOE’s Office of Nuclear
Energy, Advanced Fuels Campaign of
the Fuel Cycle R&D Program. Neutron
irradiation of Fe-Cr-Al alloys at ORNL’s
HFIR user facility was sponsored by the
Scientific User Facilities Division, Office
of Basic Energy Sciences, DOE. APT was
conducted at the CNMS, which is a DOE
Office of Science User Facility and the
MaCS Laboratory at the CAES at INL. A
portion of this work was supported by
the DOE Office of Nuclear Energy under
DOE Idaho Operations Office Contract
DE-AC07-051D14517 as part of a NSUF
experiment. The FEI Talos F200X TEM is
provided by the DOE, Office of Nuclear
Energy, Fuel Cycle R&D Program and
the NSUF. A portion of funding for SAB
was provided by the DOE Office of
Nuclear Energy’s Nuclear Energy Uni-
versity Programs.
The authors would like to thank
P.D. Edmondson and K.C. Littrell for
assistance in developing analysis tech-
niques used in this work. In addition,
they thank Y. Yamamoto for providing
the materials for irradiation and anal-
ysis, and R.H. Howard for leading irra-
diation capsule design efforts. Finally,
the authors acknowledge the staff of
the CAES, Irradiated Materials Examina-
tion and Testing (IMET) hot cell facility,
Fig. 5 —
(a) Colored STEM-ADF image, acquired on the [111] zone axis. (b) STEM/EDS map for
Cr-K
α
x-rays. (c) Color overlay of ADF image and EDS map. All images from the Fe-18Cr-2.9Al alloy
irradiated to 7.0 dpa at 320°C.
(a)
(b)
(c)