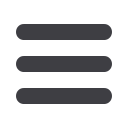
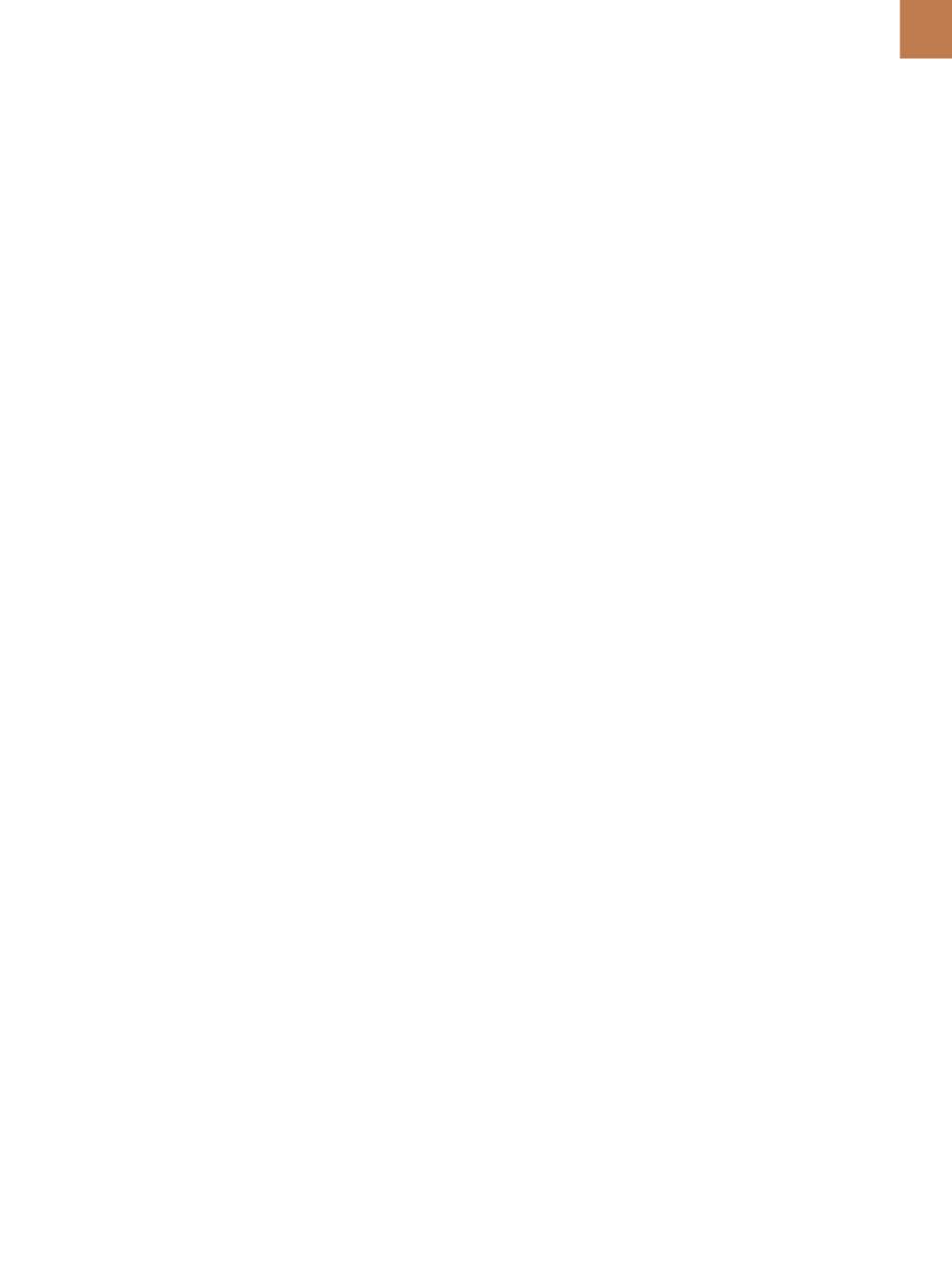
A D V A N C E D
M A T E R I A L S
&
P R O C E S S E S |
M A R C H
2 0 1 6
2 5
Diamond can exist in the interiors
of the outer planets (Uranus and Nep-
tune) and Earth’s mantle, consistent
with the phase diagram, where pres-
sure/temperature are 600 GPa/7000K
and 135 GPa/3500K, respectively.
Currently, diamond powders are syn-
thesized by graphite to diamond con-
version at high pressures and tem-
peratures. Graphite is transformed into
diamond at temperatures above about
2000K at 6-10 GPa using a liquid metal
(iron) catalyst, a process used for com-
mercial synthesis of diamond
[1]
(Fig. 1).
Due to the high binding and activation
energies of transformation, carbon
polymorphs exist metastably well into
a pressure-temperature region where
a different phase is thermodynamical-
ly stable. For example, diamond sur-
vives indefinitely at room temperature,
where graphite is the stable form.
This article discusses the discov-
ery of the direct conversion of carbon
into diamond at atmospheric pressure
and ambient temperature in air
[3-4]
. The
transformative attributes of the discov-
ery enhance diamond yield and reduce
manufacturing costs. Thermodynam-
ic limits are bypassed with the help of
kinetics to create novel carbon-based
structures with exciting new proper-
ties. Rapid melting using nanosecond
laser pulses enables modification
of the equilibrium thermodynamic
(P vs. T) phase diagram. Super-under-
cooled states of molten carbon and
BN are created, which are quenched to
form novel phases (Q-carbon and Q-bo-
ron nitride, or Q-BN) from which differ-
ent diamond and cubic boron nitride
(c-BN) structures are formed. The entire
process is completed in less than a mil-
lionth of a second, and the process can
be repeated 10 to 200 times per second.
The critical breakthrough is that
synthesis and processing are done in
a liquid phase, where diffusivities are
on the order of 10
-4
cm
2
/s
-1
, some eight
orders of magnitude faster than the
highest solid state diffusivities. This
research focuses on synthesis and
scale-up processing of nanodiamonds
(1-100 nm of uniform size), microdia-
monds (100-500 nm), and microneedles
(>2000 nm), as well as large-area sin-
gle-crystal thin films on practical sap-
phire and glass substrates, from which
diamond and related c-BN are harvest-
ed and substrates recycled. The dis-
covery provides an inexpensive way to
convert carbon into diamond and hex-
agonal boron nitride (h-BN) into c-BN
and harvest them conveniently for a
variety of applications.
RESULTS AND DISCUSSION
The atomic structure of carbon at-
oms consists of six electrons (1s
2
2s
2
2p
2
)
whose charge is balanced by six pro-
tons in the nucleus, which also has six
neutrons. Mixing wave functions of
the outer four electrons (2s
2
2p
2
) deter-
mines bonding characteristics in the
crystalline phase. The sp
2
hybridization
leads to formation of graphite bond-
ing within the sheets, and the extra 2s
electron provides for the delocaliza-
tion of electrons and metallic behavior.
The sp
3
hybridization, by comparison,
leads to the formation of diamond
semiconductor with a bandgap of
5.52 eV. Thus, transformation of graph-
ite to diamond requires controlled mix-
ing of the outer four electrons, which
has been attempted electronically us-
ing high-power photon (laser) beams
during laser ablation of graphite. Re-
sulting diamond-like carbon films have
a very high fraction of local sp
3
bonding
in the amorphous phase, but diamond
phase formation with long-range sp
3
bonding occurs only occasionally. How-
ever, providing diamond seeds enables
growing the diamond phase on the
seeds. In this transformative approach,
the 2s
2
and 2p
2
electrons are mixed
thermally. Melting rapidly creates me-
tallic super-undercooled carbon with
delocalized electrons, and quenching
achieves short-range sp
3
bonding in
Q-carbon and long-range sp
3
bonding in
diamond, which can be nucleated from
the Q-carbon, or grown on a template
directly from the super-undercooled
carbon.
Amorphous
metastable
dia-
mond-like carbon with some sp
3
con-
tent and super-undercooled liquid
carbon are introduced into the ther-
modynamically stable forms of carbon,
graphite, diamond, liquid, and vapor
[1]
(Fig. 1). This is accomplished by nano-
second laser melting of diamond-like
carbon, where the undercooled state is
at a temperature around 4000K, about
1000K lower than the melting point of
graphite. Quenching the super-under-
cooled liquid forms Q-carbon, nano-
diamond, and microdiamond by con-
trolling nucleation and growth times.
Providing a growth template enables
growing large-area single-crystal thin
films through the paradigm of domain
matching epitaxy
[7]
. The new state of
carbon (Q-carbon) has a very high frac-
tion of sp
3
bonded carbon and the rest
sp
2
, and is expected to exhibit novel
physical, chemical, mechanical, and
catalytic properties. There is more than
a 10% reduction in volume when as-
deposited amorphous carbon is melted
in the undercooled state and quenched
as Q-carbon. The Q-carbon exhibits
unique properties including ferromag-
netismat room temperature and above.
Formation of the cubic diamond phase
can occur if sufficient time is allowed
for homogeneous nucleation, or when
substrates that are lattice and planar
matched with cubic diamond are pro-
vided during nucleation
[2-4]
.
The primary focus of this work is
on synthesis and processing of nano-
diamonds, microdiamonds, and mi-
croneedles, as well as large-area single-
crystal thin films at ambient pressure
and atmospheric pressure in air. This
is achieved by nanosecond laser melt-
ing of diamond-like amorphous carbon
films on practical sapphire and glass
substrates. Irradiation with ArF Exci-
mer laser pulses (193 nmwavelength or
photon energy of 6 eV and pulse dura-
tion of 20 ns) confines laser energy and
selectively melts diamond-like carbon
films. Undercooling values for amor-
phous diamond-like carbon are con-
siderably higher than those achieved
during melting of crystalline carbon,
such as highly oriented pyrolytic graph-
ite (HOPG) samples, which do not yield
diamond
[8-9]
. Nanosecond pulsed laser
melting of amorphous carbon leads to a
highly undercooled state, which can be