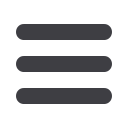
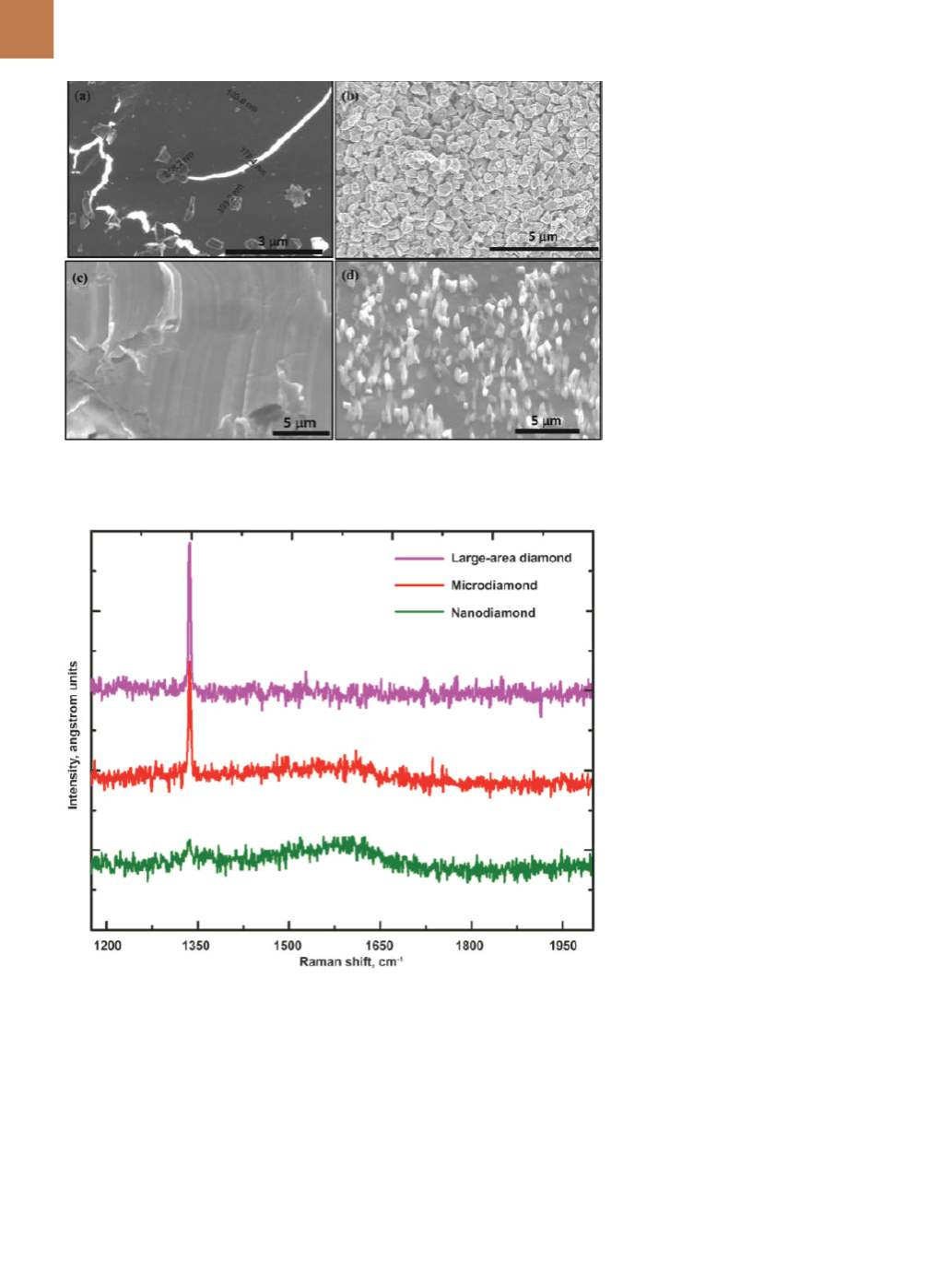
A D V A N C E D M A T E R I A L S & P R O C E S S E S | M A R C H 2 0 1 6
2 6
quenched into a new state of Q-carbon
from which nanodiamonds, microdia-
monds, and microneedles are formed.
The structures obtained from practical,
inexpensive sapphire and glass sub-
strates are conveniently harvested and
substrates recycled, thus reducing the
carbon footprint and creating very use-
ful products for mankind.
Q-carbon serves as a mother
source for diamond nucleation and
growth. Figure 2a shows the formation
of Q-carbon and growth of microdia-
mond from it after irradiation with a
single laser pulse. The Q-carbon exhib-
its a bright contrast under secondary
electron imaging in a scanning elec-
tron microscope. Kelvin probe force
microscopy (KPFM) was used to mea-
sure surface potential on the Q-carbon
filaments, which were embedded into
diamond-like carbon. Results show up
to 40 meV lower surface potential than
that of diamond-like carbon, indicat-
ing a higher field-emission potential
compared with the already inherently
high field-emission properties of dia-
mond-like carbon. Surface potential
decreases at nanodiamonds embedded
into the Q-carbon filaments, and is low-
er than Q-carbon values.
Measurements are consistent with
secondary electron-emission contrast
in the SEM images, showing significant-
ly enhanced contrast for the Q-carbon.
Controlling diamond nucleation and
growth enables converting all the car-
bon into microdiamonds (500 nm aver-
age size) as shown in Fig. 2b. Formation
of large-area single-crystal diamond
films is achieved when sapphire is pro-
vided as a template during growth from
the super-undercooled state as shown
in Fig. 2c. Formation of 200 to 300-nm
diameter by up to 3.0 µm long nanon-
eedles and microneedles is achieved
by nucleation and growth of diamond
from liquid carbon. The micronee-
dles are single crystals, and grow out
of Q-carbon near the film-substrate
interface.
Raman spectroscopy is used to
characterize diamond and related ma-
terials
[10]
. Its versatility is further im-
proved by using a range of excitation
wavelengths, particularly one wave-
length in the UV range, as the sensi-
tivity to sp
3
bonding is the strongest.
Figure 3 shows spectra from nanodia-
mond, microdiamond, and large-area
thin films. The characteristic sharp
peak at 1332 cm
-1
confirms the forma-
tion of diamond phase and the poten-
tial of the transformative technique.
The results in Fig. 3 are from 8-nm
nanodiamond, 500-nm microdiamond,
and 50+
µ
m thin film.
DIRECT CONVERSION OF
HEXAGONAL AND CUBIC BN
Direct conversion of h-BN into
pure c-BN is accomplished by nanosec-
ond laser melting at ambient tempera-
tures and atmospheric pressure in air.
Fig. 2 —
SEMmicrographs: (a) nucleation of microdiamond from the Q-carbon (mechanism of
carbon into diamond conversion), (b) mixture of nanodiamond andmicrodiamond, (c) entire
area covered with microdiamond, and (d) diamond nanoneedles andmicroneedles.
Fig. 3 —
Raman spectroscopy of nanodiamond, microdiamond, and large-area thin films using
633 nm laser excitation wavelength.