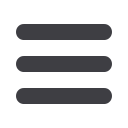
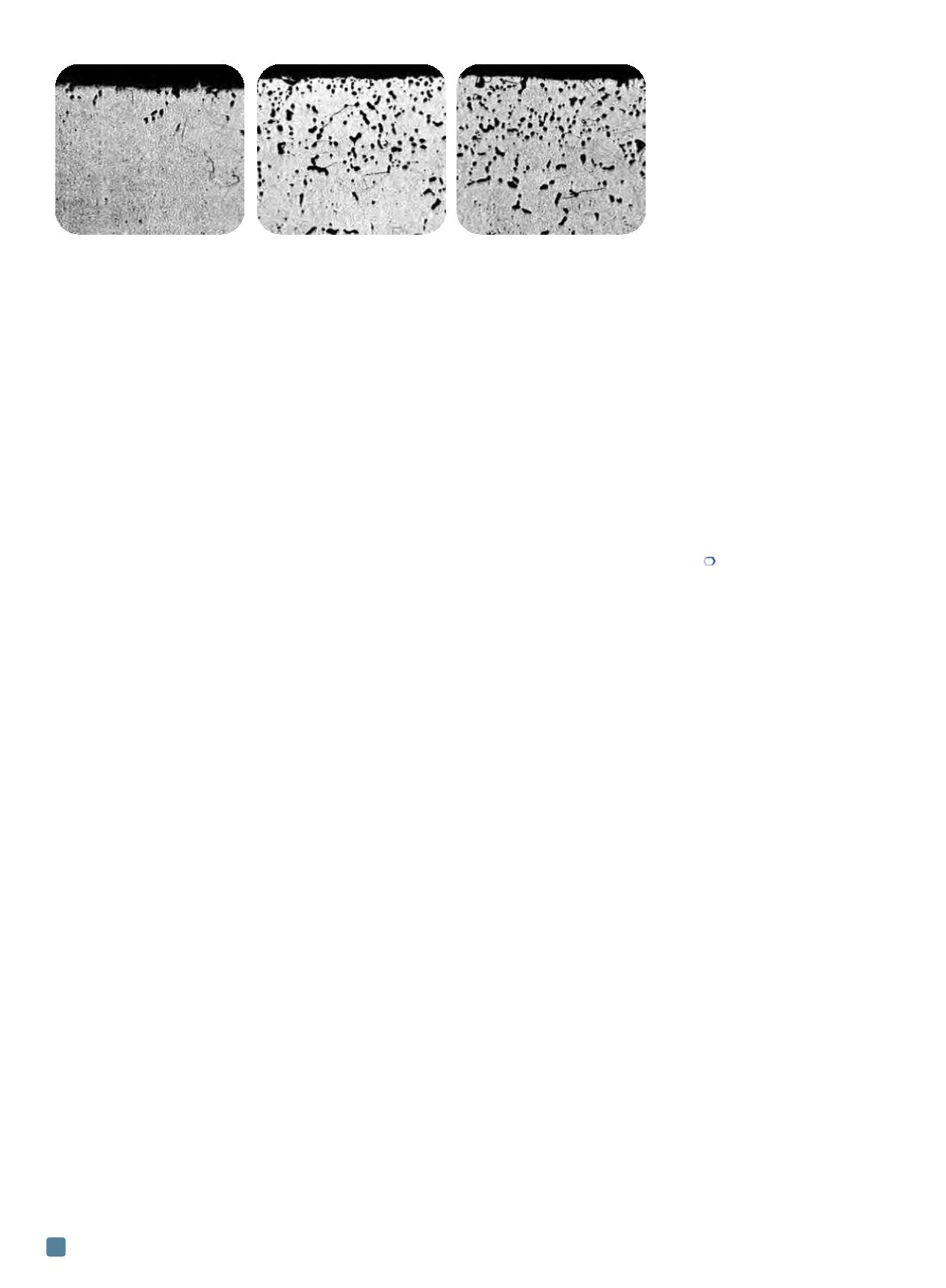
many fission products. FHR is intended for operation at
700°C or higher
[10]
. This concept originates back to research
performed at Oak Ridge National Laboratory during 1950-
70
[11]
. Under the program known as molten salt reactor ex-
periment (MSRE), a 7.5 MW molten salt reactor with
uranium fuel dissolved in a molten fluoride salt was built and
operated at 650°C. The present FHR concept uses solid
TRISO fuel particles immersed in the molten salt medium,
but many of the corrosion challenges will be similar to those
experienced in MSRE. Fluoride salt FLiBe (2LiF-BeF
2
) is
being considered as a primary coolant for FHR.
Corrosion of structural materials in molten fluoride
salt is an important issue in FHR
[12]
. In most high temper-
ature environments, corrosion resistance is achieved by
promoting the formation of a protective oxide film on the
alloy surface. However, in molten fluoride salt systems, any
such protective oxide films dissolve by the fluxing action of
the salt and cannot be used for corrosion protection. Once
the passive oxide film is removed, corrosion proceeds by
the attack of the least noble constituent of the freshly ex-
posed alloy surface. Thermodynamically, the salt fluorides
are more stable than metal fluorides, indicating that most
structural alloys should be stable in molten fluoride salts.
Ni is relatively immune to corrosion in molten fluoride
salts. Studies show that impurities such as moisture can
dramatically accelerate corrosion due to the formation of
hydrogen fluoride
[13]
. Examples of impurity reactions are
listed below. In the case of FLiBe, for example, water could
react with Be- and Li- fluorides in the salt and produce HF
gas, which in turn could leach the Cr in the alloy into the
salt as fluorides. Impurities such as Ni or Fe in the salt can
also promote dissolution of Cr into the salt. Figure 5 illus-
trates the effect of impurities in the molten fluoride salt on
corrosion from MSRE research.
BeF
2
+ H
2
O = BeO + 2HF
2LiF + H
2
O = Li
2
O + 2HF
2HF + Cr = CrF
2
+ H
2
NiF
2
+ Cr = CrF
2
+ Ni
Other types of corrosion in molten salts include dissimilar
material corrosion where deterioration is driven by ele-
mental activity differences between various materials in
the molten salt. For example, corrosion of stainless steel
tested in a molten fluoride salt in graphite crucibles is sig-
nificantly greater than in stainless steel crucibles
[12]
. Ther-
mal gradients can also drive corrosion due to the strong
dependence of solubility on temperature. Corrosion prod-
ucts that dissolve at the higher tem-
perature sections of the system can
partially plate-out in the relatively
cooler sections of the system. Cor-
rosion control in molten salts be-
gins with using high purity salts
with extensive purification treat-
ments. For FLiBe and other fluo-
ride salts, sparging with H
2
/HF is
effective for purification. Controlling the salt’s redox po-
tential by adding elements such as beryllium is also effec-
tive in mitigating corrosion
[11]
.
Hastelloy N—a relatively low Cr content alloy with
Mo—was developed by the MSRE program exclusively
for molten fluoride salt applications
[11, 13]
. This alloy must
be code-certified for use at the higher temperatures re-
quired for FHR. Currently, 316L stainless steel with ac-
tive redox control is being considered for FHR
applications. FHR will also involve other materials such as
graphite and SiC/SiC composites. Corrosion testing and
evaluation of all these materials in molten FLiBe salt at
the intended high temperatures of FHR is required and
being conducted as a part of the FHR development pro-
gram in the U.S. and China.
For more information:
Kumar Sridharan is Distinguished Re-
search Professor, Department of Materials Science and Engi-
neering at the University of Wisconsin, 1500 Engineering Dr.,
Madison, WI 53706, 608/263-4789,
kumar@engr.wisc.edu,
www.engr.wisc.edu/centers/staff/sridharan_kumar.html.References
1. Zirconium Alloys in the Nuclear Industry, 15th International
Symposium, Sunriver, ASTM International, STP 1505, 2007.
2. T.R. Allen, R.J.M. Konings, and A.T. Motta, Corrosion in Zirco-
niumAlloys,
ComprehensiveNuclearMaterials,
Vol5,p49-68,2012.
3. C.J. Wood, Water Chemistry Control in LWRs,
Comprehensive
NuclearMaterials,
Vol 5, p 17-47, 2012.
4. M. Caro, et al., Heavy LiquidMetal Corrosion of Structural Ma-
terials in Advanced Nuclear Systems,
JOM,
Vol 65, No. 8, p 1057-
1066, 2013.
5. J. Zhang, AReview of Steel Corrosion by Liquid Lead and Lead-
Bismuth,
Corros. Sci.,
Vol 51, p 1207-1227, 2009.
6. F. Barbier andA. Rusanov, Corrosion Behavior of Steels in Flow-
ing Lead-Bismuth,
J. of Nucl. Mater.,
Vol 298, p 231-236, 2001.
7. M. Shindo,WJ. Quadakkers, andH. Schuster, Corrosion Behav-
ior of High Temperature Alloys in Impure Helium Environments,
J. of Nucl. Mater.,
Vol 140, p 94-105, 1986.
8. R.Wright, et al.,HighTemperatureBehavior ofCandidateVHTR
Heat ExchangerAlloys, 4th International TopicalMeeting onHigh
Temperature Reactor Technology, 2008, Washington, D.C.,
HTR2008-58200, 2008.
9. C. Cabet andF. Rouillard, CorrosionofHighTemperatureMetal-
licMaterials in VHTR,
J. of Nucl. Mater.,
Vol 392, p 235-242, 2009.
10. C.W. Forsberg, P. Pickard, andP.F. Peterson,MoltenSalt-Cooled
AdvancedHighTemperature Reactor for Production of Hydrogen
and Electricity,
Nucl. Technology,
Vol 144, p 289-302, 2003.
11. H.G. MacPherson, Development of Materials and Systems for
Molten Salt-Reactor Concept,
Reactor Technology,
Vol 15, No. 2, p
136-155, 1972.
12. K. Sridharan and T.R. Allen, Corrosion inMolten Salts, Molten
SaltsChemistry:FromLaboratorytoApplications,ElsevierInc.,2013.
13. D. Wilson, Corrosion Issues in Molten Fluoride Salts, Ameri-
canNuclear Society Annual Conference, Reno, NV, 2006.
ADVANCED MATERIALS & PROCESSES •
JANUARY 2014
20
Fig. 5 —
Cross-sectional images of Alloy 600 after corrosion tests in fuel-containing molten
fluoride salt performed in MSRE program at Oak Ridge National Laboratory showing the effect of Ni,
Fe, HF impurities in the salt on corrosion. (a) low Fe and Ni, (b) high Fe and Ni, and (c) high HF
[13]
.
(a) (b) (c)