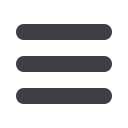
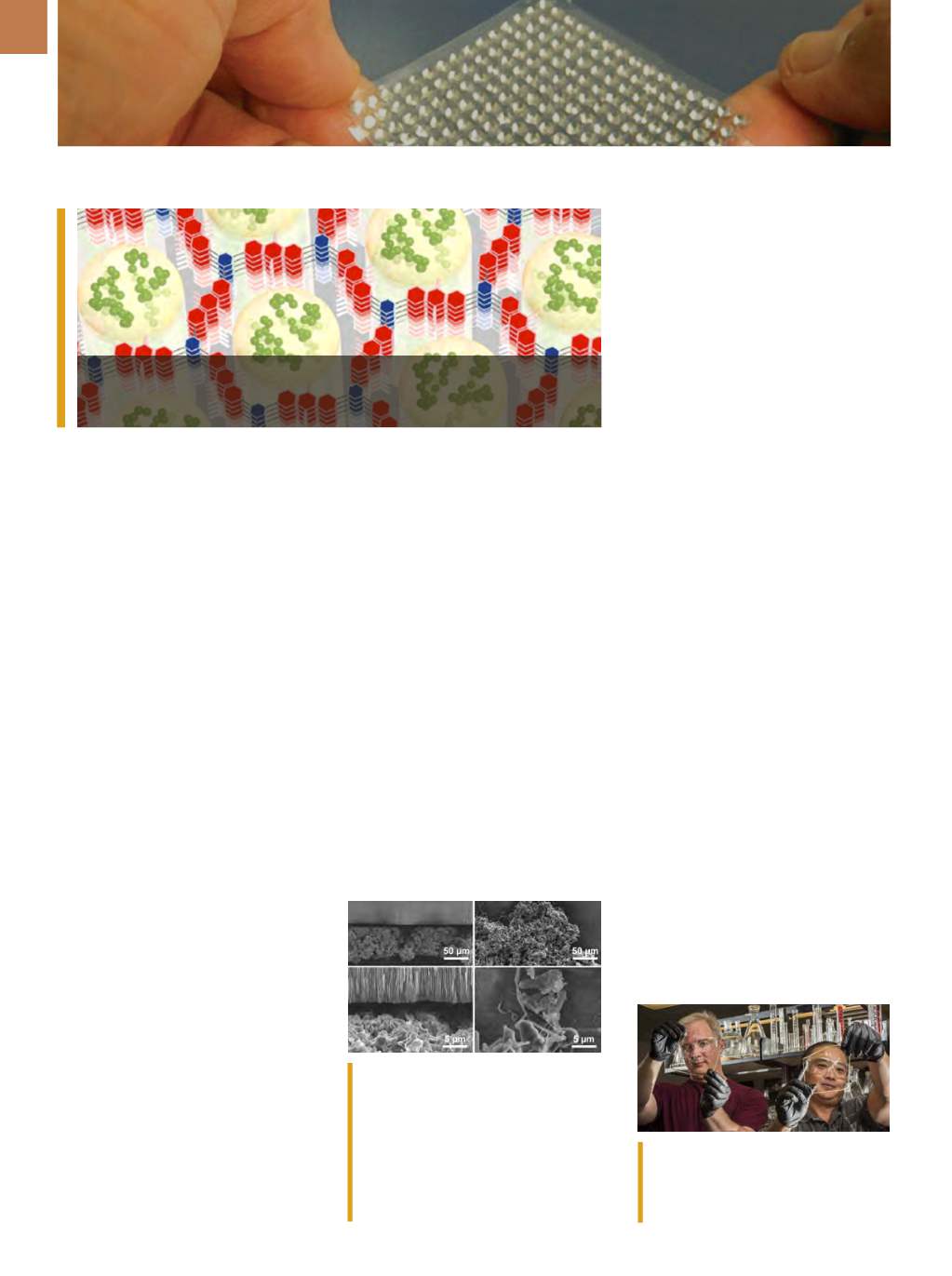
A D V A N C E D M A T E R I A L S & P R O C E S S E S | N O V E M B E R / D E C E M B E R 2 0 1 6
1 4
ENERGY STORAGE MODEL
COULD BE GAME CHANGER
A team from Cornell University,
Ithaca, N.Y., developedaway tocombine
the large energy-storage capacity of
batteries with the superior charge-dis-
charge rate of supercapacitors to come
up with a powerful electrical energy
storage device. The technology—based
on a covalent organic framework (COF)
infused with an electronically conduct-
ing polymer thin film—could benefit
numerous industries such as automo-
tive by speeding up the charging pro-
cess, extending single-charge range,
and even incorporating the device into
the body of the car itself.
The research centers on COFs—
porous crystalline structures that can
be fashioned into lightweight building
blocks with a variety of applications.
Conventional COFs have limited poten-
tial for electrical energy storage due
to their poor conductivity. The group
developed a way to overcome this lim-
itation by electropolymerizing a thin
film of poly (3,4-ethylenedioxythio-
phene) known as PEDOT (an electron-
ically conducting polymer) within the
pores of the COF, which is grown on a
conducting substrate. The resulting COF
film exhibits a 10-fold higher current
response compared with unmodified
COF films, as well as stable charge-dis-
charge for at least 10,000 cycles.
For more information: Hector Abruña,
hda1@cornell.edu,
www.cornell.edu.
METAL HAS MULTIPLE
PERSONALITIES
Battery researchers have been
focusing on lithium metal electrodes as
leading contenders for improving the
amount of energy that batteries can
store without increasing their weight.
But lithium in this metallic form has a
problem that has stymied much of this
research effort: As batteries are charged,
fingerlike lithium deposits form on the
metal surface, which can hamper per-
formance and lead to short circuits that
damage or disable the battery.
Now, a team of researchers at
Massachusetts Institute of Technology,
Cambridge, says it has carried out
the most detailed analysis yet of how
these deposits form, and reports that
there are two different mechanisms
at work. While both forms of deposits
A conductive polymer (green) formed inside the small pores of a covalent organic
framework (red and blue) works with the framework to store energy rapidly and
efficiently.
ENERGY TRENDS
On right, SEM images show two types of
lithiumdeposits, the bulky, mossy type
(top), which grows from its base, and
the needlelike dendritic type (bottom),
which grows from the tips. At left, SEM
images show the effect of a blocking layer
of ceramic material that limits growth of
mossy deposits.
are composed of lithium filaments, the
way they grow depends on the applied
current. Clustered, “mossy” deposits,
which format low rates, grow from their
roots and are relatively easy to control.
The much sparser and rapidly advanc-
ing “dendritic” projections grow only at
their tips. The dendritic type, research-
ers say, are harder to deal with and are
responsible for most of the problems.
For more information: Martin Z. Bazant,
balkwill@mit.edu, web.mit.edu.
FUEL CELL MEMBRANE
IS JUST RIGHT
Fuel cells provide power with-
out pollutants. However, membranes
in automobile fuel cells often work
at temperatures either too hot or too
cold to be maximally effective. Now a
polyphenyline membrane patented by
Sandia National Laboratories, Albu-
querque, N.M., seems to work just right,
says chemist Cy Fujimoto.
The membrane, which operates
over a wide temperature range, lasts
three times longer than compara-
ble commercial products, according
to Fujimoto and his colleagues. Their
ammonium ion-pair fuel cell—cre-
ated at Los Alamos National Labo-
ratory—combines
phosphates
with
the Sandia-patented membrane. The
ammonium-biphosphate
ion
pairs
exhibit stable performance over a wide
range of temperatures from 176°-320°F,
respond well to changes in humidity,
and last three times longer than most
commercial PEM fuel cell membranes.
sandia.gov.
Cy Fujimoto, right, and Michael Hibbs
demonstrate the clarity of their new
fuel cell membranes. Courtesy of Randy
Montoya.